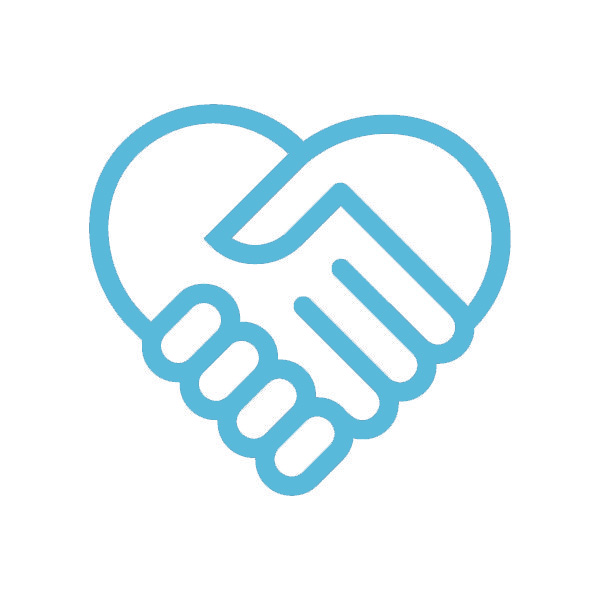
Home Care You Can Trust
We are here for you whenever you need us, for however long you need us. At Freedom Home Care and Medical Staffing, we never lose sight of the details and focus on the customer service our clients have come to expect.
Since our founding in 1997, Freedom Home Care and Medical Staffing has grown to be one of the leading and most respected in-home care providers throughout the Chicagoland, northern Illinois and North Shore area. We provide post-surgery care, Alzheimer’s care, and general in-home care services all specialized to meet your needs.